Argovia Projects 2017
PROJECT | PROJECT LEADER | COLLABORATORS | |
---|---|---|---|
12.01 A3EDPI - Applicability of 3D Electron Diffraction in the Pharmaceutical Industry | Dr. Tim Grüne (PSI) | Dr. Eric van Genderen (PSI) | |
12.04 AntibakVlies - Antibacterial equipment of nonwoven materials by means of e-grafting. | Dr. Sonja Neuhaus (FHNW Windisch) | Dr. Joachim Köse r(FHNW Muttenz) Hansjürg Härd i(Jakob Härdi AG, Oberenetfelden) | |
12.09 MicroSlide - Biomimetic microstructures to improve sliding and wear behavior | Dr. Christian Rytka (FHNW Windisch) | Prof. Dr. Ronald Holtz (FHNW Windisch) Dr. Helmut Schift (PSI) Michael Siegfried (BRUGG CABLES INDUSTRY) | |
12.10 MiPIS - Microfluidic Protein Isolation, Stabilization and cryo-EM Preparation for High-Resolution Structural Analysis | Dr. Thomas Braun (Biozentrum, Univ Basel) | Prof. Dr. Marianne Hürzeler (FHNW Muttenz) Dr. Mohamed Cham i(BioEM Lab, UBAS) Michael Hennig (leadXpro, Villigen) | |
12.13 PlasmoRetarder - Plasmonic nanoscale retarder controlled with liquid crystals | Dr. Benjamin Gallinet (CSEM Muttenz) | Dr. Yasin Ekinci (PSI) Dr. Jean Dahdah (Rolic Technologies Ltd., Allschwil) | |
12.17 3D Cellophil® membrane - 3D printable nano porose Cellophil® membranes with nano hydroxyapatite gradient for tissue regeneration applications | Prof. Dr. Uwe Pieles (FHNW Muttenz) | Dr. Stefan Stübinger (HFZ, University of Basel) Dr. Christian Geraths (CIS Pharma AG, Bubendorf) |
12.01 A3EDPI - Structural analysis with diffracted electrons
In the A3EDPI Argovia project, an interdisciplinary team of scientists from the Paul Scherrer Institute (PSI), C-CINA (Biozentrum, University of Basel), Dectris AG, and Crystallise! AG is investigating whether electron nanocrystallography can be used in pharma research to clarify the three-dimensional structure of organic agents. Dr. Tim Grüne from the PSI is leading the project, which aims to improve data collection and processing in electron nanocrystallography and clarify its relevance for developing pharmaceutical agents.
To efficiently develop new active pharmaceutical ingredients and obtain approval, researchers require the exact three-dimensional structure of the substances. If they consist of individual crystals, the spatial structure can be determined using X-ray structural analysis. In many cases, however, scientists have access only to powder, blends of crystalline grains between 10–500 nanometers in size. The A3EDPI project is now investigating whether electron nanocrystallography can be used efficiently to clarify the spatial structure of the different molecules. To do so, the samples are exposed to a high-energy electron beam. The electrons have wave properties; depending on how the atoms are arranged, a very specific diffraction pattern is produced for each molecule that provides insights into their atomic structure.
Initial experiments using a few model substances have already returned data of outstanding quality. The team is now investigating whether electron nanocrystallography can be further developed into an attractive standard for the pharma and chemical industry, and whether performance and quality requirements for industrial applications can be fulfilled.
12.04 AntibakVlies - Less bacteria on non-woven materials
In the AntibakVlies Argovia project, scientists at the School of Engineering (FHNW), the School of Life Sciences (FHNW), and Jakob Härdi AG are developing a new procedure to equip non-woven material with antibacterial and hydrophilic properties. The researchers are also working on an efficient testing method to analyze the antibacterial efficiency of the material. There are currently no materials with the desired combination of properties, which is why the project team led by Dr. Sonja Neuhaus from the Institute of Polymer Nanotechnology (INKA, School of Engineering) is aiming to modify existing non-woven materials. From previous projects, the researchers know that functionalized synthetic surfaces can be produced via e-grafting. This method involves impregnating the material with various compounds and then irradiating it with low-energy electrons. If the conditions are right, this can create a covalent and thus permanent bond. In the AntibakVlies project, different combinations of antibacterial polymers are now being examined to prevent a wide range of bacteria from growing.
12.09 Microslide - snakeskin inspires reduced friction
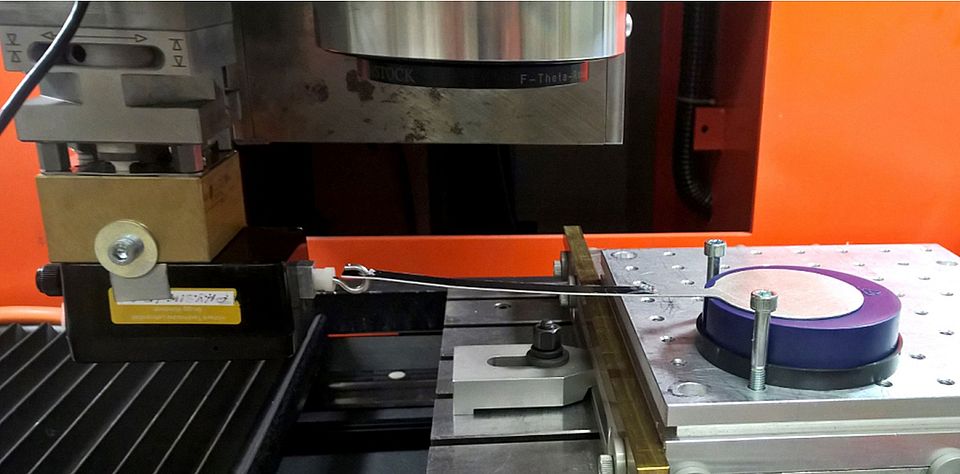
In the Microslide Argovia project, scientists from the University of Applied Sciences and Arts Northwestern Switzerland (FHNW) and the Paul Scherrer Institute (PSI) are working with Brugg Drahtseil AG (a company based in Birr) to improve the anti-friction and anti-wear properties of flat belts, as used in elevators, for example. The researchers are taking inspiration from nature; the animal kingdom offers numerous examples of special surface structures that enable gliding movement with little friction.
The team, led by Dr. Christian Rytka from the Institute of Polymer Nanotechnology (INKA), plans to apply a scale-like structure, as seen in snakes, for example, to the surface of polyurethane bands used for lifting. This structure will permanently improve anti-friction properties and therefore reduce the wear on the bands. Initially, a testing facility is to be constructed that will allow bands of various structures to be compared with those used today. The properties of different surface structures will then be examined on small areas embossed using a roll-to-roll process. Subsequently, the researchers plan to produce the first prototypes.
12.10 MiPIS - Quick protein processing for analysis
In the MiPIS project, scientists from C-CINA (Biozentrum, University of Basel) and the School of Life Sciences (FHNW) are working with their industrial partner leadXpro AG (based in Villigen) on a microfluidic system to process proteins and prepare samples for analysis using cryo-electron microscopy. The project is led by Dr. Thomas Braun (C-CINA) and builds on previous work supported by the SNI. Cryo-electron microscopy (cryo-EM) is now an established standard method for clarifying the atomic structure of complex proteins, for example to develop new active ingredients for drugs. Compared with other processes, cryo-EM requires much lower quantities of protein – just a few nanoliters – while providing precise images at atomic resolution. The traditional methods of processing proteins do not always meet the requirements of cryo-EM because they are time-consuming, require larger quantities of protein, and sometimes corrupt the spatial arrangement of the protein complexes. The scientists in the MiPIS project are now using microfluidic systems to prepare samples and hope to see major advantages over the traditional methods. A microfluidic system has already been developed at C-CINA in which a sample is placed directly on a slide required for electron microscopy. This system is now to be further developed within the MiPIS Argovia project so that proteins can be cleaned, stabilized, and prepared for cryo-EM analysis within two hours without losing their spatial structure.
12.13 PlasmoRetarder - electrically controlled colors
In the Nano Argovia project PlasmoRetarder, researchers at CSEM in Muttenz and the Paul Scherrer Institute in Villigen are working with industrial partner Rolic Technologies Ltd (Allschwil) to develop a plasmonic phase retarder which can be used for displays in sensor technology and imaging applications. The project is led by Dr. Benjamin Gallinet of CSEM Muttenz.
On the surface of nanostructured metals, electrons can be excited to collective oscillations known as surface plasmons. These plasmonic nanostructures are able to focus light at the nanoscale and to influence its color, phase and polarization. As a result, they can be used, for example, as color filters with outstanding resolution and a wide range of applications. The researchers working on the PlasmoRetarder project aim to develop, characterize and optimize a controllable phase retarder. To achieve this, they are producing nanostructures measuring up to 100 nanometers and working on an optimal coating process using metals. With the help of liquid crystals, the team hopes to be able to electrically control the color emitted by the phase retarder. In a second step, the phase retarder will be incorporated into a device intended to demonstrate the technology’s future potential in optoelectronic equipment.
12.17 3D Cellophil® membrane - patient-specific and tailored to conditions in the mouth
The Nano Argovia project 3D Cellophil® aims to develop innovative nanostructured implants that support the regeneration of bone and soft tissue in the jaw and mouth area, and can be custom-built for each patient using 3D printing techniques. Professor Uwe Pieles of the FHNW School of Life Sciences is the project leader in charge of the team, which includes researchers from the FHNW, the Hightech Research Center of Cranio-Maxillofacial Surgery at University Hospital Basel and Bubendorf-based company CIS Pharma AG.
In the project, the researchers develop a triple-layered polymer membrane based on the Cellophil® technology developed by CIS Pharma. Cellophil® is a combination of various natural amino acids linked by an acrylic backbone and characterized by a high degree of biocompatibility. The polymers are mixed with cross-linking substances. After exposure to UV light, this gives rise to membranes with varying degrees of porosity depending on the amount added. The researchers make use of this phenomenon and give the three layers of the implant different properties. The two outer layers are porous, supporting the attachment of bone-building osteoblasts to the surface facing the bone, and of fibroblasts on the side in contact with the soft tissue. The membrane’s middle layer contains a higher proportion of cross-linking substances, making it less permeable to cells. This is intended to prevent the fast-growing fibroblasts from overrunning the osteoblasts, which propagate at a slower rate, and impairing bone formation.
Furthermore, the two outer layers contain different concentrations of active substances specifically selected to support the growth and differentiation of osteoblasts or fibroblasts, respectively. Despite the different composition of the three layers, they can be individually tailored to the patient and printed in a single step.