Argovia-Projects 2023
The following projects started in 2023.
PROJECT | PROJECT LEADER | COLLABORATORS |
---|---|---|
| ||
18.01 CAPOFOX - CAPillary Optics for FOcusing of X-rays | Dr. Helmut Schift | Dr. Sina Saxer |
18.05 NanoFemto Tweezers - Multi-Celular Tissue Assembly with Holographic Optical Tweezers Based on Diffractive Nano-Optics and Femtosecond Red Diode Pumped Alexandrite Laser | Prof. Dr. Bojan Resan | Dr. Maurizio Gullo |
18.06 NanoHighSens - NanoHighSens: Nanoscale and High Bandwidth Current Sensor | Prof. Dr. Joris Pascal | Prof. Dr. Stefan Gorenflo |
18.07 QSBI - Quantum Sensors for Brain Imaging | Prof. Dr. Kirsten Moselund | Dr. Moritz A. Kirschmann |
18.08 SmartCoat - Innovative SmartCoats for efficient and targeted delivery of RNA-based | Prof. Dr. Johannes Mosbacher | Prof. Dr. Georg Lipps |
18.01 CAPOFOX — Smooth mirrors for X-rays
As part of the Nano-Argovia project CAPOFOX, an interdisciplinary team is working on the further development of lithographic techniques. The researchers from the Paul Scherrer Institute PSI, the School of Life Sciences at the University of Applied Sciences Northwestern Switzerland FHNW and the industrial partner XRnanotech aim to produce micro-optical components with very low surface roughness from polymers. With this in mind, they are looking for a way of producing tiny capillary mirrors that are suitable not only for ultraviolet radiation but also for X-rays.
Polymer structures can be produced on a large scale with various designs and are therefore seeing increasing applications in optical components. Moreover, grayscale lithography can be used to produce elements with specific three-dimensional contours — including lenses with continuous and stepped surfaces, such as those used in smartphone cameras.
Here, following further processing, differences in lateral exposure yield different layer thicknesses of the “photoresist” — a light-sensitive material used in photolithography. Primarily for technical reasons, this method produces a small number of grayscales and therefore leads to stepping. The resulting surface roughness represents a significant limitation when it comes to applications in lenses and mirrors.
Even tiny structures cause interference
In optical components for short-wave electromagnetic waves in the range of extreme ultraviolet light (10 to 121 nm) and X-rays (under 10 nm), even tiny steps on a similar scale to the wavelengths cause interference, causing unwanted scattering as waves reflect off the surface.
In previous Nano-Argovia projects, the team working under project leader Dr. Helmut Schift (PSI) developed various methods for localized surface smoothening. These contactless polishing techniques level out surface roughness using localized melting, but they cannot be used for extensive structures with longer steps such as those needed in capillary optics.
Step-free surfaces
Now, in the Nano-Argovia project CAPOFOX (CAPillary Optics for FOcusing of X-rays), which was launched in early 2023, researchers are combining tool-, laser-, design- and material-related aspects to develop a method for producing an extended 3D polymer structure such as that needed in the case of narrowing capillaries. The team’s initial focus is on producing stepless semicylindrical depressions and developing the basis for measuring nanoroughness by various techniques. At a later stage, they aim to produce a concave mirror for extreme ultraviolet wavelengths by equipping a polymer film with multiple layers to form a reflective mirror. These results will facilitate the development of ultrasmooth mirrors for X-ray focusing, with potential applications in areas such as material processing.
“The CAPOFOX project brings together outstanding expertise in the preparation and examination of ultrasmooth surfaces. The chosen methodology is innovative and is also remarkable due to its numerous high-impact future applications. We’re very much looking forward to accompanying and supporting these developments.”
Dr. Florian Döring, CEO and founder of XRnanotech
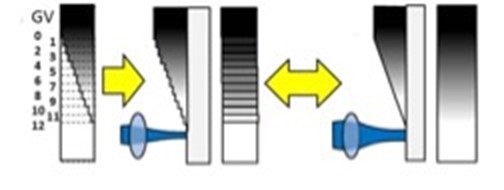
Researchers can use lithographic techniques to produce three-dimensional contours in a “photoresist.” Given that conventional methods result in stepping and therefore the unwanted scattering of light, the team working on the Nano-Argovia project CAPOFOX plans to produce structures that are smooth down to the nanometer level. (Image: PSI)
18.05 NanoFemto Tweezers – Optical tweezers for 3D printing of cells using an efficient femtosecond laser
In the Nano-Argovia project NanoFemto Tweezers, an interdisciplinary team is developing optical tweezers that can be used to assemble different cell types, including neurons, in a tiny area. For this purpose, the teams from the School of Engineering and School of Life Sciences at the University of Applied Sciences Northwestern Switzerland FHNW as well as the industry partner TLD Photonics, are using femtosecond lasers and nano-optical elements to build optical traps (tweezers). With the developed setup, the researchers plan to 3D print cells and to build organs on a micrometer surface (body-on-chip) in order to be able to study, for example, the effects of drugs on different organ systems on a chip.
The effects of pharmaceutical substances can be studied quite early on in their research and development phase, on biomedical probes (chips) where reactions of entire organs can be simulated. To investige side effects, however, it is also desirable to place not only cell types of one organ system, but of completely different organs on a chip (body-on-chip) and to reflect a broader spectrum of pharmacological effects. Given the increased development of personalized medicine, such studies could be carried out with patient-specific cells. In addition, such biochips promise the further reduction of animal experiments.
Traps for different cell types with novel efficient femtosecond lasers
The team in the Nano-Argovia project NanoFemto Tweezers, led by Professor Bojan Resan (FHNW School of Engineering), plans to develop optical tweezers that can be used to build body-on-chip systems.
Optical tweezers use a focused laser with a specific intensity profile. The laser light exerts a force on microscopic objects that causes the object – in this case, cells – to be repeatedly drawn into the focus of the laser beam, allowing it to be trapped, fixed or controllably moved.
In order to arrange different cell types on an area of just a few micrometers, the different cell types must first be “trapped” using nano-optical traps. They can then be assembled into a three-dimensional complex tissue while the process is monitored and navigated with a multiphoton microscope using the same femtosecond laser.
The researchers are initially investigating different nanolithographic methods to produce tailor-made nano-diffractive optics for traps for different cell types. Another focus is on using a laser that, with sufficiently short pulses, does not generate a lot of heat around the cell and thus does not damage the cells.
The project team is therefore developing a novel laser based on red laser diode pumped alexandrite that generate pulses in the femtosecond range (1 femtosecond = 10-15 seconds). The laser used feature high wall-plug efficiency, are compact, reliable and inexpensive. They can also gently trap cells without heating the surrounding area.
In a case study, the researchers plan to use a prototype of the optical tweezers to assemble a neural tissue.
“The Nano-Argovia project NanoFemto Tweezers allows us to investigate and commercialize one of our novel lasers for multiple new applications – optical tweezers, multiphoton imaging and micro-scale 3D printing of biomedical tissues.”
Stephan von Wolff, CEO TLD Photonics AG
18.06 NanoHighSens – Novel current sensor fulfills the latest quality standards
In the Nano-Argovia project NanoHighSens, researchers from the Schools of Life Sciences and Engineering at the University of Applied Sciences Northwestern Switzerland FHNW are collaborating with the company Camille Bauer Metrawatt AG to develop a novel current sensor. The team is using an array of eight small magnetometers that surround the current conductor, each based on 100 Magnetic Tunnel Junctions. The sensor aims to outperform existing technologies in terms of bandwidth and resolution and is expected to meet new standards for power quality measurement devices.
In the Nano-Argovia project Nanocompass, launched in 2021, the interdisciplinary team led by project leader Professor Joris Pascal (School of Life Sciences FHNW) has already successfully demonstrated that nanoscale Magnetic Tunnel Junctions are ideally suited as measuring devices for magnetic fields.
Magnetic Tunnel Junctions consist of two ferromagnetic layers separated by a thin insulating layer. When the magnetization of the two ferromagnetic layers is parallel, electrons can “tunnel” through the insulating layer more easily than when the magnetizations are in the opposite direction. This mechanism is influenced by the strength of the magnetic field that is applied to the junction. This makes the magnetic field measurable. Since current also generates a magnetic field, measuring the magnetic field generated by the current flowing in a conductor allows the strength of the current to be determined.
High resolution and wide bandwidth
In the NanoHighSens project, Joris Pascal’s team now plans to use the Magnetic Tunnel Junctions (MTJ) to develop precise measuring devices for power grids. To do this, the researchers are using eight magnetometers based on MTJs, which are arranged around a primary conductor that carries the alternating and direct current to be measured.
Each of the eight magnetometers consists of an array of 100 nanoscale MTJs, including their interconnection, arranged on an area of 100 μm x 100 μm. Since the respective measurements are based on an average of 100 magnetic field measurements, this results in very good resolution with an improved signal-to-noise ratio compared with conventional devices.
The high bandwidth of MTJs – the amount of data that can be transmitted – also promises advantages over other approaches.
As part of the Nano-Argovia project, the project team will test different Magnetic Tunnel Junctions and the design of the new current meter and validate them at Camille Bauer Metrawatt’s facilities.
“The NanoHighSens project is an essential basic building block for a holistic energy efficiency assessment taking into account the power quality footprint.”
Max Ulrich, Managing Director, Camille Bauer Metrawatt AG
18.07 QSBI – Quantum sensor for diagnostics in the brain
In the Nano-Argovia project QSBI, an interdisciplinary research team is investigating the possibility of using quantum sensors based on nitrogen vacancy centers in diamonds to study brain activity. The team, which includes researchers from the Paul Scherrer Institute PSI, CSEM Allschwil and the industrial partner Qnami, plans to use the project to optimize the existing method of magnetoencephalography. In addition, the researchers aim to develop an algorithm so that a three-dimensional map of brain activity can be created from the measurement data.
When nerve cells communicate with each other, weak electrical currents flow and magnetic fields are generated that can be measured. Magnetoencephalography (MEG) uses magnetic field sensors to analyze these magnetic fields in the brain. The method contributes to the better understanding of brain activity, as billions of nerve cells interact with each other, and opens the door for treatment possibilities.
Innovative combination
The team, led by Prof. Kirsten Moselund of the Paul Scherrer Institute PSI, together with Dr. Moritz Kirschmann from CSEM and Dr. Tobias Sjölander from the quantum sensing company Qnami now aims to develop a new generation of magnetoencephalography systems that combine quantum sensors and artificial intelligence.
The researchers plan to use diamonds with nitrogen-vacancy centers (NV centers) as quantum sensors. NV centers are formed when a carbon atom in the diamond’s crystal lattice is replaced by a nitrogen atom and a carbon atom is missing at the adjacent lattice position. Single electrons orbit in these vacancy centers and their spin changes when they are exposed to an electric or magnetic field. The electrons can be excited and then emit individual light particles (photons), which provide information about the state of the spin and thus provide information about the electric and magnetic fields. Qnami has already commercialized this technology for material science microscopy applications. The existing product uses NV center probes to scan over the surface of 2D materials to map out magnetic fields on the nano-scale.
However, the brain’s magnetic fields are very weak and are easily disturbed by magnetic fields in the environment. Therefore, to achieve this ambitious goal the researchers combine their interdisciplinary skills to use the PSI nanofabrication facility to produce diamond based photonic crystal structures that improve the signal-to-noise ratio of the signals generated by the NV centers. They also plan to exploit the established expertise at CSEM to develop machine learning algorithms that can process the data and eventually lead to the reconstruction of a 3D brain activity map with high accuracy and robustness.
Key advantages
The envisioned quantum sensors would have major advantages over conventional systems. They can operate in room temperature and don’t require cryogens. Further they could measure signals from the brain without being in a magnetically shielded room. They could be integrated into headgear, could also measure brain activity during various activities and would be suitable for children.
“We believe that due to their combination of robust operation, easy logistics and high sensitivity, NV diamond magnetometers will revolutionize biomagnetism.”
Dr. Tobias Sjölander, Quantum Engineer at Qnami AG
18.08 SmartCoat – Innovative approach in the treatment of cancer
In the Nano-Argovia project SmartCoat, researchers are investigating a novel method to downregulate specific genes that drive proliferation and malignancies in cancer. The researchers from the School of Life Sciences FHNW, the Paul Scherrer Institute PSI and industry partner Palto Therapeutics are using nanoparticles to transport specific short fragments of RNA (siRNA). The nanoparticles also ensure that the siRNA is only taken up by targeted cancer cells, where it can reduce the activity of specific genes.
The use of siRNA (small interfering RNA) is currently being investigated as a highly specific method to treat diseases characterized by overproduction of certain proteins.
There are, however, some challenges in the therapeutic use of siRNA, some of which have not yet been solved. The siRNA has to reach and enter the diseased target cells without being blocked by organ barriers in our body, nor being enzymatically degraded or excreted. In addition, healthy cells should not take up the siRNA to avoid undesirable side effects.
Nanoparticles into cancer cells
The team in the Nano-Argovia project SmartCoat, led by Professor Johannes Mosbacher (FHNW), is now testing a novel approach to shuttle siRNAs to specific target genes that play a crucial role in various aggressive tumor types such as ovarian and pancreatic cancer.
The researchers are using bioengineered nanoparticles called SmartCoats to deliver the siRNA. The SmartCoats act as a physical barrier. They protect the siRNA from enzymatic degradation and from interactions with immune cells. Their specific design also ensures that the siRNA is taken up only by targeted cancer cells. These cancer cells have specific structures (shuttling receptors) on their surface that enable binding and subsequent uptake of the SmartCoat-siRNA complexes into the cell. In this way, the RNA fragments only enter the degenerated cells and can exert their regulatory effect there.
The project team will produce the SmartCoat-siRNA complexes in the team of Professor Georg Lipps (FHNW) using a method developed by Palto Therapeutics and subsequently characterize them structurally and biophysically in the team of Dr. Roger Benoit (PSI) with the goal of improving their physico-chemical properties and stability.
The researchers also plan to use in vitro cellular assays with timelapse imaging to optimize the efficacy of the SmartCoat-siRNA complexes. Palto Therapeutics will use these results to turn these complexes into effective drugs.
“The ongoing project with FHNW and PSI is a cornerstone of the innovation of Palto Therapeutics.”
Dr. William L. Wishart, Director Palto Therapeutics Inc.