Applications
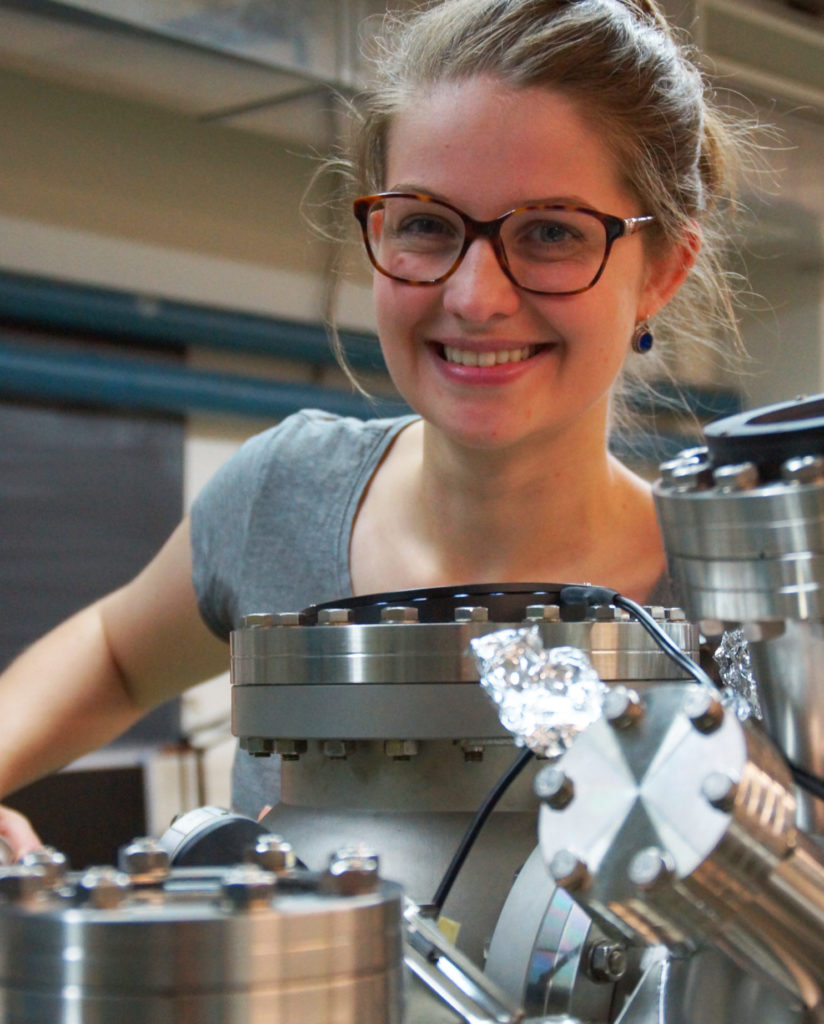
The nanosciences could not develop until new ways of exploring the world of atoms and molecules had become available. In 1981, Heinrich Rohrer and Gerd Binnig took the first step by developing the scanning tunnel microscope (STM). This microscope works by having a probe – usually in the form of a sharp tip – scan the surface of an electrically conductive sample line by line. The tunnel current is measured for every point scanned on the sample surface, indicating the distance from the tip. The readings obtained in this way are processed on a computer and put together as a digital image, yielding a profile of the surface.
In 1986, the atomic force microscope (AFM) was unveiled by Gerd Binnig, Christoph Gerber and Carl Quate. It enables non-conductive samples, too, to be examined in precise detail at atomic resolution. These microscopes, known collectively as scanning probe microscopes, have been developed in many different ways since then, allowing for a diverse range of applications.
Observing natural systems
Now it is possible to image biological samples at atomic resolution, and even to show in moving pictures the activity of the natural structures within living cells. Argovia Professor Roderick Lim’s group is studying nuclear pore complexes, for example, which regulate the transport of chemical compounds in and out of the cell nucleus. The team has come up with a new theory on how nuclear pore complexes work, using film footage to corroborate this (Research group Roderick Lim).
Making the tiniest forces visible
In physics, too, different groups are using scanning probe microscopes to investigate physical phenomena in detail and to improve our understanding of them. For example, Professor Ernst Meyer’s group has measured the very weak Van der Waals forces between individual atoms for the first time. These forces operate between non-polar atoms and molecules. Although they are very weak by comparison with chemical bonds, they play a significant role in nature. They are important in all processes involving grip, adhesion, friction or condensation; for example, they explain why geckos are such skillful climbers (http://www.nature.com/ncomms/2016/160513/ncomms11559/full/ncomms11559.html).
Back in 1986, the Nobel Prize Committee acknowledged the invention of the scanning tunnel microscope by awarding the Nobel Prize to Binnig and Rohrer. Thirty years later, in 2016, the development of the atomic force microscope was also recognized by the awarding of a prestigious prize. Christoph Gerber, Gerd Binnig and Carl Quate received the Kavli Prize because, according to the accompanying press release from the Kavli Foundation, atomic force microscopy is “a powerful and versatile scientific technique that continues to advance nanoscience for the benefit of society” (https://www.unibas.ch/en/News-Events/News/Uni-People/Christoph-Gerber-to-Receive-the-Kavli-Prize.html).
Using electrons to achieve better resolution
The development of electron microscopy has also made a key contribution to study of the nanoworld. An electron microscope uses electrons to image the object that is being examined. As an electron beam has a much shorter wavelength than light, electron microscopes can achieve much better resolution (up to less than a nanometer) than light microscopes.
The development of electron microscopy goes back to 1931, when Ernst Ruska and Max Knoll built the first transmission electron microscope (TEM). This groundbreaking development earned Ernst Ruska the Nobel Prize in 1986. Since then, electron microscopes have made huge strides. Today, there is a wide range of electron microscopes available that can image both surfaces and internal structures in precise detail.
Testing and using new miracle materials
Nano scientists all over the world are also testing new materials with a wide range of potential uses, thanks to their special properties. One such miracle material is graphene. This consists of just a two-dimensional layer of carbon atoms, arranged in the shape of a honeycomb. Graphene is an excellent conductor of electricity and heat. It has a tensile strength 300 times greater than that of steel, but it is also flexible and transparent.
Graphene was first produced in 2004 by two scientists, Konstantin Novoselov and Andre Geim. The method they used was astonishingly simple. It involved using Scotch tape to peel off a layer just one atom thick from a graphite crystal. In 2010, they were awarded the Nobel Prize for Physics for this discovery.
Nowadays, thin sheets of graphene can be produced, cleaned and stretched in the laboratory, allowing researchers to study graphene’s special properties. Using these new methods, scientists in Professor Christian Schönenberger’s group have discovered, for example, that graphene conducts electrical current with almost no resistance, making it a very attractive option for a large number of applications (https://www.unibas.ch/en/News-Events/News/Uni-Research/Graphene-Research-Electrons-Moving-along-Defined-Snake-States.html). Other groups have established that graphene causes almost no friction. This means that it would cut energy loss in machines dramatically, if used as a coating (https://www.unibas.ch/en/News-Events/News/Uni-Research/Graphene-Slides-Smoothly-Across-Gold.html).
Learning lessons from nature
Many researchers in nanosciences take nature as their model and seek to make use of the insights that this provides. Thus Professor Cornelia Palivan’s research group is looking at artificial membranes with a similar structure to those of natural cells. The researcher use these artificial membranes to produce tiny nanoparticles. The particles can be packed with drugs or their precursors, which are released only when they reach the site where they are needed. In this way, both side-effects and the quantities of drugs administered could be reduced significantly. The artificial membranes can also be made to incorporate natural proteins that, for example, allow water to pass through, but block salts dissolved in it. The hope is that it will be possible to desalinate water economically using this kind of approach.
One large field where nature often serves as a model is that of structured surfaces. Nowadays micro and nanofabrication techniques can be used to alter such surfaces specifically to prevent dirt or microorganisms from sticking to them. These methods enable textiles, for example, to be made dirt-resistant or equipped with cooling properties. Other instances include the production of artificial bones. Here researchers are trying to modify the surface to provide ideal conditions for bone-forming cells, making it as easy as possible for them to grow into the piece of bone replacement material. Teams of scientists all over the world are also looking at ways of using nanostructures on surfaces to create security features.
Using artificial atoms to produce a quantum computer
One major area of nanoscience that is continually hitting the headlines is the development of a quantum computer. Professor Daniel Loss’s team has suggested using the spin of individual electrons to do this. According to his theory, artificial atoms (quantum dots) could be used to produce a quantum computer. However, there are still many experimental problems that need to be solved before the theory can be put into practice.
Innumerable questions and applications
There are many more possible applications for nanoscience. Nanoscientists are consulted, for example, when people are looking to improve the use of solar energy, are developing new sensors, are seeking faster and more efficient methods of data transmission or are looking to develop completely new materials.
The fundamental science projects underway at the SNI’s PhD school form the basis for new discoveries at the SNI. To look at specific applications of nanoscience, every year the Nano Argovia program provides funding to about 10 applied research projects run in partnership with industrial companies from Northwestern Switzerland.
You will also find many examples of the SNI’s research and its possible applications in SNI press releases and the SNI’s annual report.